Geology: Introduction and Essential Concepts
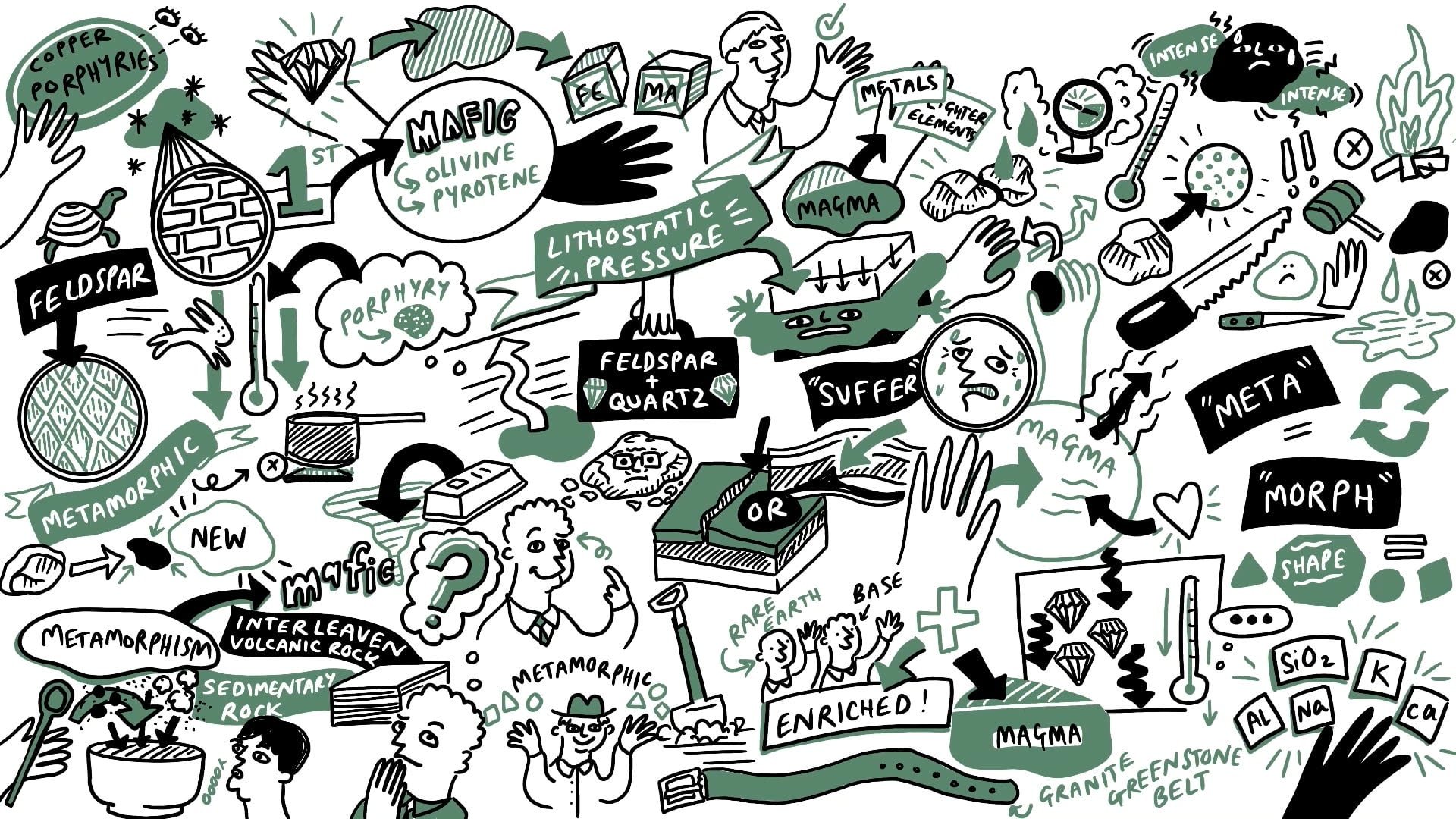
More episodes
Transcript
Today I’m going to be talking about geology, which is a subject very close to my heart. This is going to be fun!
Well, fun as it may be, it has also been hard for me to put together because I’m essentially trying to condense one of my favourite subjects into manageable, digestible chunks of information. Preparation for this part of The Con has proved to be a useful (painful?) exercise in synthesis and precis.
I have channelled my inner Winston Churchill "If I had more time I would have written you a shorter letter" and tried to make it as concise as possible, but the geology section will nevertheless run for several episodes. Short, but still not that short.
I went back to episode 9 and listened to the three minute introduction to geology, and decided to expand significantly on the content that I initially planned to include. Feedback from listeners to The Con series so far has made me realise that there is appetite for me to deal with complex subjects thoroughly. And that is exactly what I’m going to do here in this geology section of The Con, I’m going to address Geology thoroughly but with necessary omissions and simplifications.
Firstly, The Con is not an academic course, and these few episodes on Geology are not an undergraduate degree in Earth Sciences (even if they do contain a few more practical points... but let’s not go there). Ore deposit geology is complex and as a field it draws on many of the concepts and principles of geology that are taught at undergraduate level. One of the reasons I loved it at university was because to be good at it you needed to be good at a whole suite of specific geological disciplines. And that’s still the case. Successful exploration and deposit evaluation requires a wide and deep range of understanding. And, yes, I still love it.
This ‘long-introduction’ episode, therefore, will be dedicated to introducing general geological concepts that are useful for understanding economic geology. You may be glad to hear, however, that I will not be attempting to squeeze a full degree into the podcast. There will be no tests at the end.
Three subsequent episodes will deal with the following topics:
The Con 34 will address the question of “What is Ore?” Background crustal abundances of elements (and minerals). Concentration factors required to create ore. Different types of orebody. Capital Expenditure requirements, barriers to entry, and which kinds of companies are suited to which kinds of deposits.
The Con 35 brings it all together in a discussion of Mineral Systems - the holistic approach to exploration and mineral deposit evaluation. A discussion of the six key components that need to be in place in a Mineral System for deposits to be formed, plus a quick nod to the concept of Mineral Endowment and Metallogenic Potential.
Finally, The Con 36 will be an exercise in Jargon busting. Having worked your way through Cons 33 to 35, this episode will be a summary geological section covering language, terms, geological features and deposits, that are frequently used in news releases and technical presentations. It will also provide a whistle-stop tour of some of the junior market go-to deposits.
For now, however, as Dylan Thomas once said... “to begin at the beginning”.
You’re familiar with the main concepts, right? A mineral is a solid chemical compound of a given composition and crystal structure that occurs naturally, and rocks are simply an assemblage of minerals. There are three broad families of rock types, Sedimentary, Igneous, and Metamorphic.
Sedimentary rocks are accumulations or depositions at surface, with erosion, gravity, wind, water, frost, and evaporation important agents in their formation. These accumulations are typically preserved over geological time by being buried and protected from erosion. During burial sediments can undergo a number of different processes such as compaction, diagenesis, and cementation all of which get lumped together in a process called lithification. Sometimes young rocks are barely compacted or cemented at all. So sedimentary rocks are both an accumulation of sediments and then that sediment pile being turned into actual rock (with vary degrees of lithification).
A key feature of mineral deposits that we will explore in a later session is the source of mineralising fluids. Note that water trapped in the sedimentation process is called connate water, and that this water plays an important part in geochemical processes when it is heated up, squeezed out of the rock, moves around, and reacts with other rocks. More on this in the Mineral Systems episode.
Igneous rocks (from the latin word for fire, ‘ignis’) are formed from the cooling of magma or lava. Nice and simple. As well as classifying igneous rocks on their chemical or mineralogical composition, they are also classified by crystal size. The faster the molten rock cools, the smaller the crystal size and the inverse is true as well. The slower it cools, the larger the crystal size. Magma that makes it, or extrudes, to the surface as lava usually presents itself in volcanos, and cools quickly. These are called extrusive or volcanic rocks and are typically relatively fine-grained. Magma that accumulates in a magma chamber within the earth’s crust simply intrudes into existing rock and forms plutons, and cools slowly. These are called intrusive or plutonic rocks and are typically relatively coarse-grained. I told you it was easy.
Sticking with magmas for a bit, because it’s relevant to mineral deposits... A feature of a cooling magma chamber is something called crystal fractionation. In the early 1900s Canadian petrologist, Norman Levi Bowen, established that as a melt cools, crystals start forming in a predictable pattern.
The first crystals to solidfy and form are mafic minerals such as olivine and pyroxene that are rich in magnesium and iron. Mafic - the ma from magnesium, the fe from ferric. Anyway, once the first minerals crystallise, it leaves the rest of the melt relatively depleted in iron and magnesium, and relatively enriched in the other elements such as aluminium, sodium, calcium, potassium, and silica. Gradually as the temperature falls and more crystals form and sink as solids, the upper portions of the magma chamber can be further and further enriched in elements stable at lower temperatures. And these elements enriched in magma chambers can include base metals and rare earth elements.
(only draw the ‘a’ picture, and it wouldn’t be paleodepth, it would just be depth... thanks!)
Sometimes, if new forces are applied to the magma chamber (a fresh pulse from below, or volatile degassing in the magma, perhaps), lithostatic pressures (the weight of the rock mass above) can be overcome and the upper portion of the magma chamber, rich in metals and lighter elements, possibly carrying some feldspar and quartz crystals, can rise up higher in the crust as part of a fresh intrusion. Remember that a porphyry is a fine-grained intrusive rock (in other words it has cooled quite quickly) that also contains well defined, larger crystals of feldspar that formed more slowly in a hotter place. Hello copper porphyries, yes, I’m looking at you.
But that’s enough detail for now. Next up are metamorphic rocks.
The process of metamorphism does not melt rocks, but instead transforms pre-existing rocks into denser, more compact rocks. New minerals are created either by rearrangement of mineral components or by reactions with fluids that enter the rocks. Pressure or temperature can even change previously metamorphosed rocks into new types of metamorphic rocks. It is fair to say that metamorphic rocks experience a range of intense conditions, and a very common verb in geological text is “to suffer”. As in, metamorphic rocks often suffer, deformation / shearing / smearing / folding. Despite these hot and pressured conditions, metamorphic rocks do not get hot enough to melt, or they would become igneous rocks. The etymology of metamorphic is simple: ‘meta’ means change and ‘morph’ means shape.
Conditions capable of stiumulating metamorphism are most often found either deep in Earth’s crust or at plate boundaries where tectonic plates collide. As much of our continental crust is old, and has been buried, so there are plenty of metamorphic rocks in the day-to-day life of an exploration geologist. And indeed in the life of a resources investor.
Granite greenstone belts anyone, and all those gold deposits? Have you ever wondered what makes a greenstone belt? Well, they are extensive layers of interleaven mafic volcanic rock and sedimentary rock (a volcano-sedimentary sequence or complex), that have been subject to metamorphism. And it just so happens that the classification of metamorphic rocks is largely based on the presence of identifier minerals in the mix... an observable mineral assemblage, which is predictable in time and space and becomes known as a mineral facies.
Mafic rocks (rich in iron and magnesium) when metamorphosed to a certain grade can reform into a suite of green minerals that includes chlorite, actinolite, and epidote. Do you see where this is going? Yep, you got it, greenstones. And these volcano-sedimentary packages are variously intruded by granitic plutons, plugs and stocks, which are perhaps themselves some of the drivers of metamorphism alongside burial and compaction. Hence “Granite Greenstone Belts”
This is, of course, a necessary simplification as the Greenstone mineral facies can be formed as the rocks are rising in temperature and pressure (prograde metamorphism) or when falling in temperature and pressure (retrograde metamorphism). Very old rocks can suffer several prograde and retrograde episodes, which makes it complicated trying to work out when the most economically important mineralisation event occurred, and metals can be remobilised and later fluid pulses can overprint primary mineralisation with secondary mineralisation, and multiple phases of alteration... Und so weiter... it can make your head spin. Unpicking the history of rocks is akin to a detective trying to establish what happened on the night of the crime using forensic science. No PC Plods please, bring us your finest geoscientist.
A final word on metamorphic rocks is that in the process of compaction and rearrangment into denser forms, metamorphism can generate fluids. This metamorphic dewatering can mix with connate brines released during sedimentary compaction, and possibly even with devolatalising magmatic fluids... so the systems and sources of fluids can get pretty complex, hot, and acidic. Complexity worthy of a degree course and a further lifetime of study by experts (not me, I’m far too much of a generalist). And of course these fluids can all carry metals, which again will be addressed in the Mineral Systems episode of The Con.
Are you still with me? Sedimentary, check. Igneous, check. Metamorphic, check.
Right. Now, let’s put these key rock types into context, and explore some other concepts such as pressure, temperature and density.
We live on a roughly spheroidal planet that formed 4.6 billion years ago, and I’d like you to cast your mind back to happy memories of Year 6 geography lessons with Mrs Bigginsthwaite. Unless you are really really old you were probably shown a diagram a bit like this... Here, let me draw it for you
It’s the classic plate tectonic diagram showing an oceanic spreading ridge (constructive plate margin) in the centre. Here the hot magma upwells and comes into contact with seawater. The magma cools to form oceanic crust which, thanks to the chemistry of the seawater, is slightly different chemically from the solid rock of the lithosphere underneath. Oceanic crust is usually about 7 km thick, but can be thinner when the plates are spreading more slowly, and thicker - such as in Iceland where oceanic crust thickness is up to 20 km thick - where the spreading is happening faster.
The molten magma forms a distinctive suite of rocks in the ocean, that includes sheeted dykes and - pillow lavas, where each gloop of lava forms a quenched, fine-grained skin on contact with water and the rest of the hot bubble cools more slowly, with the coarsest crystals in the middle.
Looking deeper into the earth, a couple of really cool things can be pointed out here... Firstly, the lithosphere and the aesthenosphere are the same rocks in terms of composition, it is just that under huge pressure and at high temperatures, the aesthenosphere is just not as solid as the lithosphere. How is your Greek? Litho means “rock”, “Aesthenes” means weak. The aesthenosphere flows (over geological time) like a slightly plastic, very viscous rock. It is ductile. In contrast the lithosphere is essentially brittle, and it can crack and form fractures. Together these two spheres form the upper mantle, a section of the earth largely rich in magnesium and iron silicates.
This concept is especially important for mineral deposit formation. Rocks that are chemically identical can behave very differently when subject to different temperatures, and different pressures over time. Ductile or plastic rocks can flow and deform, due to the temperature or pressure conditions. When those same rocks are at lower pressures and temperatures, they can be brittle or inelastic and then break and fracture. Remember most deposits like fractures as these fractures and faults act as permeability pathways and concentrate fluid flow. Also, most deposits like heat engines driving circulating fluids and hydrothermal processes. So both environments (cool and brittle, and hot and ductile) have their role to play in mineralizing processes.
To make things even more complex, a rock that is buried quickly will experience pressure instanteously, but it might take tens or possibly hundreds of millions of years to reach thermal equilibrium with its new location.... By which stage it might have been brought up to the surface again and be experiencing lower pressures already, but just reaching it’s peak temperature. Crazy, right?
I hope that you will be seeing by now that the pressures involved, and the temperatures, and the time scales are immense. Away from the spreading centre, the lithosphere is about 100 km thick, plust the 7 or so km of oceanic crust on top. Unimaginable thicknesses, forces, and pressures! You’ve all dived down to the bottom of a deep swimming pool, right? What’s that? Maybe 5 meters? And you can feel the pressure...from all sides.
Coming back to the main picture, oceanic crust is largely made up of magnesium-rich and iron-rich minerals, mirroring the composition of the mantle, - mafic or ultramafic. It has a density of 3 g/cm3. In comparison the density of continental crustal rocks is about 2.7 g/cm3. Pure water at room temperature and pressure has a density of 1 g/cm3, and under the same conditions, gold has a density of about 19.3 g/cm3, copper 8.9 g/cm3, and aluminium 2.7 g/cm3, the same density as continental crust.
Density contrasts are helpful in the exploration phase as geophysicists can identify denser formations such as the presence of ultramafic bodies or indeed large accumulations of dense sulphide (metalliferous) minerals - which will show up on a gravity survey. Miners also need to have very accurate density measurements of any given resource and surrounding material to understand how many tonnes of rock and waste might need to be moved.
The iron-rich minerals formed at an oceanic spreading centre also faithfully record the magnetic signature of the earth at the time of emplacement. Given that the earth’s polarity undergoes mysterious magnetic reversals irregularly (between 10,000 and 50,000,000 years, with the last one almost a million years ago), magnetic surveys of the oceans reveal the growth of oceanic crust over the eons in great detail. Incidentally, all iron-rich minerals that are common in mafic and ultramafic units retain remnant magnetism, which means that magnetic surveys used by exploration geophysicists can help map geological units at depth without drilling expensive holes.
But I am getting distracted. Back to the picture.
To the left of the spreading centre that are mantle plumes, hot spots, a subduction zone and a volcanic island arc. Underwater volcanic activity will be mixed with sedimentation and in the subduction zone, sediments and seawater will be trapped in an accretionary wedge, and subducted, and eventually melted when it gets deep enough. Further to the left in the picture you can see a volcanic arc, and sediments accumulating in the subduction zone are part of the fore-arc basin, and sediments accumulating behind the volcanic arc are part of the back-arc basin. If it helps to visualise modern examples, The Aleutian Islands off the coast of Alaska and the Lesser Antilles south of Puerto Rico are good examples volcanic island arcs forming above the subduction zone between two oceanic plates.
To the right of the spreading centre, the oceanic crust and the attached lithosphere is being subducted beneath a continental plate where the crust is typically 40 km thick, on top of the 100 km thick lithosphere. The subduction of an oceanic plate beneath a continental plate has even more sediment loaded into the basin as there are more bits of broken rocks (clasts) coming from Terra Firma (terrigenous)... so plenty of terrigenous-clastic sediment that is loaded into the trench, piling on weight and sediment into the basin, which then gets incorporated into the accretionary wedge. These sediments, plus the water, and the oceanic crust eventually melt, and like a lava lamp rise in vast plutons and magma chambers into the mountains adjacent to the subduction zone. The archetypal example of this setting are the Andes mountains of South America. In addition to llamas and pan-pipes, you get volcanos, earthquakes, and occasionally mineralised porphyries.
A vast array of sedimentary process will also be taking place along this coastal mountain chain. We can zoom in on the main depositional environments
In high energy environments with steep slopes, and fast flowing water sediments can be chaotic with large boulders or cobbles incorporated in the mix. I’m thinking erosional sloughing off of high mountains, flash floods, and collapses off the steep continental shelf into the deep ocean.
In low energy environments such as wind-blown plains, meandering rivers, inland seas and lakes, and deep oceans, sediments accumulate slowly with well-ordered, predictable grain sizes. In river, desert and tidal environments regular winnowing by wave or wind action can sort sand or mineral grains incredibly effectively, so that everything within a certain energy environment has the same physical characteristics (governed by properties such as density, surface area, weight, hardness, or friability) But just up the beach, say, are larger stones that only get moved by storm surges. This is how mineral sands and placer deposits are enriched to the point that they might be economically viable.
On the far right of the picture is a rift zone where crustal thinning occurs, geothermal gradients increase, and a combination of subsidence, volcanism and heating occurs. Sometimes the rifting process stalls such as the formation of the North Sea between mainland Britain and Continental Europe [I’m speaking of recent geological history of course, not of recent politics .... ahem... enough of that].
An example of an active geological rift is the East African Rift. In hotter climates evaporation of water during the day may cause chemical precipitation of vast thicknesses of evaporites to accumulate (especially when accompanied with ongoing subsidence). Whether marine or lacustrine, evaporites need a restricted water balance, and can form economically important resources. Chlorides, sulphates, borates, and carbonates, give us vital salts, fertilizers, detergents, and supplements. Evaporites also play important roles in trapping fluid flow and potentially as being a repository for nuclear waste.
The final point I would like to make about this simple picture of the crust but which spawns so much complexity is about uranium. I was blown away by this when I heard about it in my very first lecture at university on the age and provenance of the Earth.
Our planet is warm and pleasant - even though at times it can feel too cold, too hot, or too cloudy yes, Canadians, Australians, and Brits, I’m thinking of you. The pleasant conditions we enjoy on its surface are in large part due to the radioactive processes taking place at its centre. The radioactive decay mostly of isotopes of uranium, thorium and potassium causes our planet to behave like an immense hot-water bottle: slowing down the cooling rate and consequently making it habitable. About half of the heat necessary for our survival is released by the radioactive disintegrations which take place in the rocks that form our Earth crust.